Relation of Adult Neurogenesis in Alzheimer’s Disease: A Review on Past, Present and Future Implications
Mainav Purohit and Chaitanya Raulji*
Division of Biomedical and Life Sciences, School of Science, Navrachana University, Vasna-Bhayli Road, Vadodara-391410, Gujarat, India.
Received: 1 July 2021 Revised: 9 November 2021 Accepted: 22 December 2021 Published: 10 February 2022
*Corresponding Author: chaitanyaraulji123@gmail.com
Abstract
Alzheimer’s disease (AD) is a neurodegenerative disease attributed to the loss of neurons causing memory loss and cognitive decline. Many low-grade neurological insults are associated with AD but the ones that have been mostly studied are pathological build-up of amyloid protein ß and hyperphosphorylation of microtubule associated protein tau. Adult neurogenesis is the phenomenon of formation of new neurons in healthy brain via neural stem cells (NSCs). Loss of extrinsic signaling (physical activity, dietary intake) results in impaired neurogenesis during AD, making it a probable biomarker for AD. The alteration of proliferation, differentiation, migration, and integration processes are involved in neurogenesis is clearly evident during AD. Thus, the possible targets for the therapeutic purpose of AD, are interleukin-4 (IL-4), brain-derived neurotrophic factor (BDNF), interleukin-6 (IL-6), fibronectin type III domain – containing protein–5 (FNDC5) which helps to improve the proliferation and integration of the neural stem cells. Studies carried out in mice models suggest an intrinsic and extrinsic correlation of NSCs for the treatment of AD with an aim to reverse the neurodegeneration. The present review focuses on the novel markers like DCX, NeuN, Sox2, calbindin and Nestin involved in adult neuronal development and its role in pathophysiology of AD. Moreover, this review opens up new avenues for treating AD and developing molecular markers for it.
Keywords
Alzheimer’s, amyloid ß, adult neurogenesis, therapeutic, biomarker
Introduction
Alzheimer’s disease (AD) lays its origin from 1906 where Dr. Alois Alzheimer first identified Alzheimer’s disease in a female patient named Auguste Deter 1 . Epidemiologically, AD affects approximately 5 million people in the US and this number is expected to rise by 35 million by 2050 2. In 2015, India reported 4.1 million cases of people living with dementia. Dementia affects 2.7 percent of the population in India, according to epidemiological studies conducted between 1996 and 2006, with Alzheimer’s disease being the most common cause (1.3%) 3. It was estimated in 2010 that 36.5 million people were living with dementia, with 7.7 million new cases each year and a new case of dementia every 4 seconds 4. The World Health Organization (WHO) estimated that 0.379% of the world’s population suffered from dementia in 2005 and its prevalence will increase to 0.441% in 2015 and 0.556% in 2030 5. Histological characterization of AD revealed extracellular deposits called cerebral plaques in neocortex and hippocampal region and are made up of a thick proteinaceous core containing the amyloid β (Aβ) peptide surrounded by dead and weakened neurons. The other histopathological hallmarks are the filamentous, hyperphosphorylated form of the microtubule-associated protein tau which forms neurofibrillary tangles in neurons of the same regions of the brain 6. Although most cases of AD occur occasionally, about 5% of patients develop an early disease as a result of a completely penetrant autosomal dominant gene mutations (APP, APOE, PSEN1, PSEN2) 6. Till now, evidence pertaining to the specific remedies in preventing Alzheimer’s disease remains elusive 7.
As discussed, AD mostly affects the hippocampal area in the brain8. Hippocampus is one of the areas in the adult brain where evidence of neural stem cells (NSCs) are documented 9. Adult NSCs are capable of producing new neurons throughout life in two major parts of the adult mammalian brain: the subventricular zone (SVZ) of the lateral ventricle and the sub granular zone (SGZ) in the dentate gyrus (DG) of the hippocampus 10 this process is called adult neurogenesis. This process consists of different stages such as neural stem cell proliferation, lineage differentiation, migration and the integration of the developing neuron in the pre-existing circuit of the brain. Adult neurogenesis is related to the physiological functioning of the brain and degeneration. Zhao et al (2008) observed changes in the pattern of neurogenesis which were associated with many neuropsychiatric disorders.
In most cases adult neurogenesis is reduced during AD which is found in SVZ and SGZ. Concurrent studies show a dilemma in the decrease/increase neurogenesis in the brain of mice and human subjects affected with AD 11,12. Furthermore, reduction of new born neurons causes cognitive defects in AD mice 13. In line of this, the present review discusses the use of impaired adult neurogenesis in AD as a possible potential therapeutic biomarker for predicting the progression and effective treatment for the disease.
Pathophysiology of Alzheimer’s disease
The early onset of familial AD is due to genetic mutations in the amyloid precursor protein (APP), presenilin proteins – PSEN1 and PSEN2 14. Apolipoprotein E (ApoE) is the strongest genetic risk factor for sporadic AD 6. Alzheimer’s disease (AD) is thought to occur when increase in the abnormal amounts of Aβ accumulate extracellularly forming amyloid plaques. Tau proteins accumulate within cells causing the formation of neurofibrillary tangles in the brain, affecting neuronal function, connectivity and causing progressive brain function loss 15 16. Moreover, microscopically the main attribute of AD is the loss in the cerebral cortex of neurons and synapses and the hippocampal portion of subcortical regions due to accumulation of misfolded Aβ and tau proteins 17,18,19. These losses result in deterioration of the temporal and parietal lobes and severe atrophy in the affected area, including parts of the frontal cortex and cingulate gyrus. Studies using MRI and PET show that the size of affected brain regions in AD patients decreases as they progress from mild to severe cognitive impairment as compared with healthy older adults 20. Aβ plaques are formed when amyloid precursor protein (APP) undergoes complex sequential proteolytic processing in the CNS through two main processing pathways called amyloidogenic and non-amyloidogenic processing pathways 21. This APP which is a transmembrane protein is encoded by APP gene which has also gained attention due to high rate of mutation. Thus, misfolded plaques containing small peptides of 39-43 amino acids long are called beta-amyloid (Aβ). APP is important for nerve cell growth, survival, and damage recovery 22,23.
Several enzymes are known to cleave APP at several amino acid positions near C terminal. For instance, α-secretases acts on APP, 83 amino acids from its carboxyl terminus, which belongs to ADAM protease family (“A disintegrin and metalloproteinase”). Whereas, the β-secretase enzyme contains two or more different complexes called BACE1 and BACE2 which cleaves APP at 99 amino acids from its carboxyl terminus. The third type of APP cleavage is provided by the enzyme complex γ-Secretase. Presenilin-1 and Presenilin-2 mediate the catalytic function of γ-Secretase and is encoded by PSEN1 and PSEN2 gene. APP is cut twice by γSecretase generating a 50 amino acid peptide which consists of the APP C-terminal end, and is named as the amyloid intracellular domain (AICD). The second γ-secretase fragment is slightly variable, but tends to be located at 57, 59, or 61 amino acids at the C-terminus of APP. Sequential processing with α- and γ secretases yields a large N-terminal peptide called soluble APPα (sAPPα) and a smaller 3kDa peptide called P3. β-secretase cleavage produces a large Nterminal peptide called soluble APPβ (sAPPβ) and a smaller C-terminal fragment called CTFβ. The γ-secretase cleaves CTFβ, and the Aβ peptide. Depending on the continuous activity of βγ-secretase, the exact cleavage site of γ-secretase varies, resulting in Aβ peptides, which are usually 38-43 amino acids in length 24. The α-secretase enzyme in the “nonamyloidogenic pathway” appears to exhibit neuroprotective activity by cleaving APP at the Aβ sequence and releasing the sAPPa fragment through the membrane 6. Primarily in the hippocampus, neurofibrillary tangles consisting of paired helical filaments are present in the cytoplasm of neurons 25.
Neurofibrillary tangle formation in AD is directly related to protein dysfunction. Tau is a microtubule-associated protein that plays an important role in supporting axonal transport and promoting cellular stability. The main polypeptide of the paired helical filaments is the microtubule-associated protein tau. Tau levels in the AD neocortex are 7 times higher than in the control aged brain group, and this elevation is a result of abnormally phosphorylated proteins (Iqbal and Grundke-Iqbal, 2007). Hyperphosphorylation of τ reduces the binding of τ to microtubules and disrupts subsequent axonal transport 26. Abnormally hyperphosphorylated tau has shown to have a neurodegenerative effect by inhibiting microtubule function, impairing axonal transport of neurons, and enhancing functional toxicity by forming paired helical filaments 6. Apart from phenotypic markers, others like inflammatory processes, cytokines and growth factors (BDNF) are attributed to play a role in the pathophysiology of AD. Tissue damage is marked by an elevated immune response resulting in inflammation at the affected site which are considered to be the signs of secondary damage to the tissue 27. Similarly, changes in the distribution of various neurotrophic factors such as the brain neurotrophic factor (BDNF) and the expression of its receptors have been found to be associated with AD 28,29.
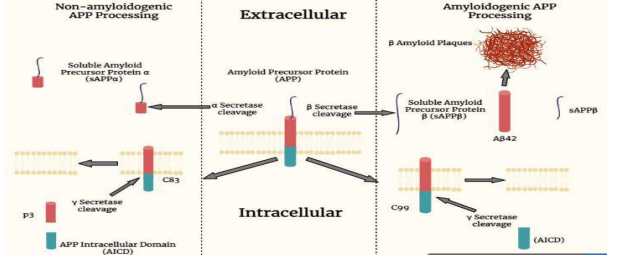
Figure 1: Generation of soluble APP peptides (sAPPα, sAPPß) is processed due to the cleavage by α-secretase, ß – secretase or γ – secretase. The C-terminal membrane anchored fragment (C83) yields P3 peptides by γ – secretase depicting the major secretory pathway which is non-amyloidogenic. The presenilin/nicastrin-mediated γ – secretase processing yields amyloid beta proteins, amyloid-beta 42 (Aβ42) from C99 fragment. Aβ42 is the major component of amyloid plaques.
Adult neurogenesis in the healthy brain
Traditionally, it was believed that no new neurons are produced after birth 30. But in the late 50s new method on labeling techniques was developed for dividing cells with [H3]-thymidine, that incorporates into the replicating DNA during the S-phase of the cell cycle, and can be detected with the help of autoradiography 31. Generation of new neurons has already been reported in the embryonic stages of mammalian CNS by Cajal et al, while using the above mentioned technique pioneering work done by Altman and colleagues demonstrated the adult neurogenesis in rats various brain regions, which includes the dentate gyrus 9, neocortex 32 and olfactory bulb 33. Advancement in staining has been done by 34 where they have used BrdU a synthetic thymidine analogue, for the detection of neuronal proliferation in mammals.
Adult neural stem cells (NSCs) are responsible for the generation and differentiation of new neurons, which were first isolated from the adult CNS of rodents 35 and later from humans 36. Combined retroviral-based lineage tracing and electrophysiological studies provided the most convincing evidence so far that newborn neurons in the adult mammalian CNS are continually produced throughout adulthood 37,38,39. However, human adult neurogenesis is currently under investigation and evidence marks the formation of new neurons throughout the human life, nevertheless the rate of production is too low compared to other mammals 40,12.
NSCs reside in two major parts of the adult brain: the subventricular zone (SVZ) of the lateral ventricle and the sub granular zone (SGZ) in the dentate gyrus (DG) of the hippocampus 41. These NSCs can self-replicate and differentiate into multiple neural lineages such as neurons, astrocytes, and oligodendrocytes 10.
Based on three major factors like morphology, molecular markers and proliferative behavior, two types of neural stem cells were identified. The SGZ of hippocampus contains type 1 neural progenitor cells (NPCs) also abbreviated as radial like neural stem cells (rNSCs), contains radial projections that spans entire granule cell layer and ramifying in the inner molecular layer of DG. They can be detected by the molecular markers such as Glial fibrillary acidic protein (GFAP), Sox2 and Nestin. These stem cells are quiescent but gets activated due to environmental factors that generate the type 2 NPCs. These cells express Sox2, Nestin, Tbr2 and MCM2 except GFAP. These cells generate both astrocytes and DCX expressing neuroblasts that migrate into the granular cell layer and undergoes maturation. These maturing/surviving cells receive inputs from the entorhinal cortex (EC) and send axonal projections through the hilus towards the CA3 area of the hippocampus 42,43. These newborn immature granular neurons in the DG receives GABAergic synaptic input around one week after birth followed by glutamatergic inputs by two weeks, and full maturation is achieved within 4 weeks in development 44. Mature granule cells mostly become glutamatergic dentate granule cells (DGCs) and express neuronal nuclear antigen (NeuN) and calbindin 43,42.
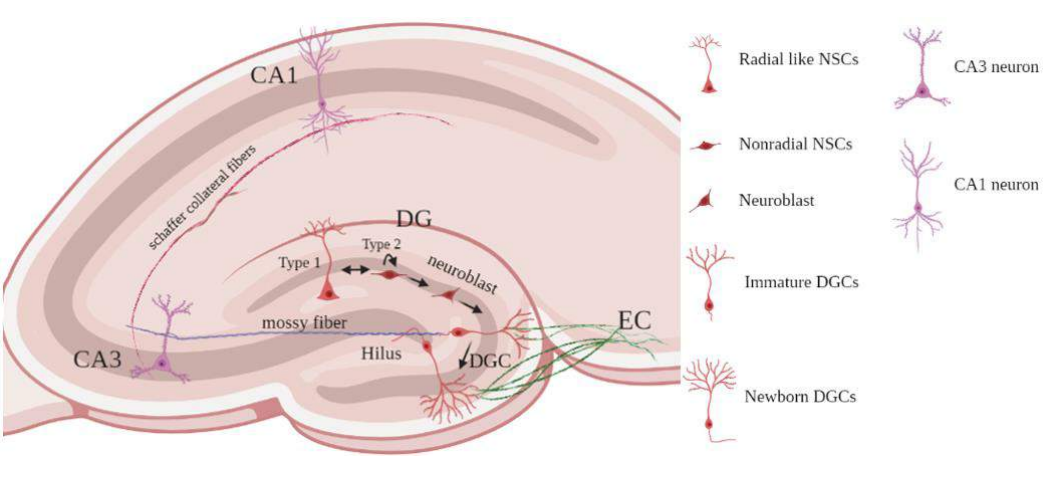
Figure 2: Neurogenesis in adult mammalian hippocampus: Radial-like neural stem cells (Type-1) which are quiescent stem cells that produce non-radial precursor cells (Type2) which are actively proliferating and produce astrocytes and neuroblasts; Neuroblast cells migration into the granular layer; Differentiate into dentate granule cells; Maturation of Newborn dentate granule cells takes place and they receive EC input and send their axons to hilar interneurons, mossy cells, and CA3 pyramidal neurons.
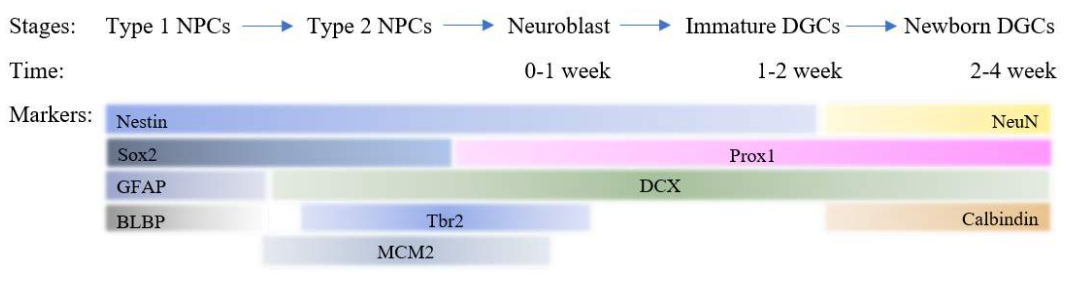
Figure 3: Stage specific markers of neuronal development in adult neurogenesis depicts time specific differentiation.
Adult neurons generated in these two neurogenic areas are integrated into existing neuronal circuits39. New adult neurons have a high input resistance and a subthreshold Ca2+ conductance, which enables new neurons for action potential firing with very small excitatory currents. This unique development may allow them to integrate easily into a mature brain without altering existing cognitive processes44. This integration plays important functions such as the migration of new neurons born in the SVZ migrate to the olfactory bulb via the rostral migration stream (RMS) and are encapsulated by the complex network of astrocyte tunnels in rodents 10,45 and in humans 46. Decreased adult neurogenesis in SVZ in mice has been reported to induce abnormal olfactory and sexual behavior47. New neurons born in the SGZ are integrated into the DG neural circuitry, which plays an important role in short-term memory generation. Impaired adult neurogenesis of SGZ in mice has been reported to impair new memory formation48,49. In particular, these new neurons are important for spatial memory formation50. Furthermore, new integrated neurons in the DG have also been important in memory consolidation during Rapid eye movement (REM) sleep in rodents 51.
Adult rats produce approximately 9,000 new neurons daily in the SGZ, with a survival rate of approximately 50%52. In an adult human, the data showed that 700 new neurons are added to the SGZ every day. About as many are also lost, keeping the total number of hippocampal cells roughly constant. The annual yield is almost 2%53.
This process of neural stem cell proliferation, lineage differentiation, migration and integration of the developing neuron in the adult brain has been regulated by both intrinsic, genetic, epigenetic factors and extrinsic signaling pathways 54. Membrane-bound extracellular factors and their intracellular signaling cascades have been identified in the regulation of the SVZ and SGZ neurogenesis, which includes Wnt, sonic hedgehog (Shh), Notch, BMPs, neurotrophins, growth factors (BDNF, FGF-2), various neurotransmitters (glutamate, GABA and dopamine), cytokines (IL-6), and hormones (estrogen, corticosterone) 44;54. Further, intrinsic mechanisms including miRNAs, transcription factors and epigenetic regulators have been shown to be crucially involved in regulating neurogenesis in the adult mammalian brain 54. Thus, future research trends will be based upon modulation of these factors, manipulating the production of new neurons, and improving the disease condition.
Association of Adult neurogenesis and AD
Adult neurogenesis reduces during normal aging in healthy brains and during Alzheimer’s disease (AD). Hippocampal adult neurogenesis helps in cognition and memory formation 55. Aging is the greatest risk factor for AD. The reduction of new born neurons during aging and AD leads to cognitive loss and impairment of memory 56. The integration of the new neurons is impaired during aging. This is because of loss of extrinsic signals (physical activity, dietary intake) or reduced response of the precursor cells/stem cells to normal signalling during aging, or AD 57.
Adult neurogenesis of both SVZ and SGZ are affected during AD. The alteration of neuronal proliferation, differentiation, maturation and survival in both SVZ and SGZ is observed indifferent transgenic adult mouse models of AD42, in human iPSC cell lines 58, and clear reduction shown in the post-mortem of AD patients compared to healthy donors 59,13. As discussed earlier there is a lack of evidence in the increase or decrease of new neurons in AD patients and some show increase in the generation of new neurons 43,60,61. This alteration in outcome may be related to the observation of different stages of AD in relation to neurodegeneration. According to various hypotheses, enhanced new born neurons may arise in the affected brain as a homeostatic self-healing mechanism62 ; and decreased neurogenesis might be contributing to the AD pathophysiology63,64. So, in the decreased condition of neurogenesis in AD, Amyloid-beta (Aβ) peptide, would deregulate new born neurons for facilitating disease progression 63. The mechanisms of how the various Aβ species affect adult neurons remain unclear, and defining the pathophysiological environment of the AD brain remains an area of research.
By studying and targeting the molecular players involved in the different stages of adult neurogenesis it can be used as a potential biomarker for AD and creating an alternative therapeutic option for the treatment. Current therapeutics for AD only provide symptomatic relief but doesn’t reverse the damage of neurodegeneration. Adult neurogenesis in the hippocampus could be an option to reverse the process of neurodegeneration. But scientists are still figuring out at what point in the disease, adult hippocampal neurogenesis plays a role in AD’s pathophysiology. Also, studies show in rodents, there is no negative impact of increased neurogenesis in AD models42, but there might be ethical concerns in humans. Adult neurogenesis is restricted in humans but there is a possibility to induce human neural precursor cells to generate new neurons, to cure neurodegenerative diseases, such as AD 11. We can enhance neurogenesis either by stimulating the production of endogenous NSCs by manipulating the extrinsic and intrinsic signals or by providing exogenous NSCs. For instance, transplanting stem cells into the AD mouse model reversed cognitive deficits 65. Additionally, more research is conducted to understand this stem cell therapy carried out in clinical trials pertaining to humans. Whereas, endogenous therapeutic targets would be to neutralize intracellular Aβ oligomers in the adult NSCs by using gene therapy, and at the same time restoring functional neurogenesis 11. Another target to cure AD through adult neurogenesis could be interleukin-4. Studies have shown that interleukin-4 inhibits Aβ42 aggregation and restores the proliferative and neurogenic ability of NSCs by suppressing Aβ42 in zebrafish 66. Other therapeutic approaches like increasing adult neurogenesis with the help of different modulators had neuroprotective effects on mice with cognitive impairment 67, so modulating neurogenesis could be helpful in reversing the process of AD. Studies show that the help of running/exercise promotes the rapid integration of new neurons in the aging brain through modulating neurotrophins, which promotes the plasticity of aging networks in the hippocampus 56. In AD condition exercise also helps in enhancing neurogenesis and improved cognition along with reduced Aβ load and also increased levels of brain-derived neurotrophic factor (BDNF), interleukin-6 (IL-6), fibronectin type III domain – containing protein–5 (FNDC5), and synaptic markers which helps to improve adult neurogenesis survival and maturation 68.
Future directions:
The alteration of adult neurogenesis during AD, could be proposed as biomarkers for AD progression in humans, and new literature indicates impaired neurogenesis in the early stages of AD patients, i.e. prior to amyloid plaque formation, so it would be of potential to use adult neurogenesis as an early stage biomarker for AD 11,59. Although AD is a multifactorial disease whose phenotypic characteristics revolves around the neuronal death. Multiple protein aggregates in the different stages of AD maybe a connecting link in neurogenesis impairment. Thus, by using advanced techniques like NGS, immunohistochemical localization and electrophysiological recordings will open new avenues for the treatment of AD. Hence, more research should be focused on extrapolating and unravelling the mechanistic base and targeting its therapeutic biomarker.
Conclusion
Current therapies for AD aim at symptomatic relief and are futile once neurodegeneration crosses a certain stage. Adult neurogenesis is a well-examined phenomenon and is still under exploration as a therapeutic mode for neurodegenerative diseases. Artificial induction of neurogenesis can be a much efficient treatment mode for AD as it can overcome the loss of neurons caused due to neurodegeneration and replenish the source of healthy neurons. However, further research is required to study the effect of induction of neurogenesis on an aging brain and its feasibility as a biomarker for Alzheimer’s disease.
Glossary:
β-amyloid protein– Peptides of 36-43 amino acids in size that cause plaques in brains of people having Alzheimer’s Disease.
Microtubules– polymers of tubulin protein that form cytoskeleton to support the eukaryotic cell.
Tau protein– microtubule associated proteins which are known to maintain stability of axons.
Neural stem cells– self-renewing cells in the nervous system that give rise to neurons and glia.
Dementia– A group of thinking and social symptoms that interferes with daily functioning.
Autosomal– located on one of the non sex chromosomes.
Cognitive– Involved in gaining knowledge and comprehension.
Familial– Family-related or occurring within a family.
Atrophy– Reduction in size of cell, organ, or tissue, after attaining its normal mature growth.
Neurotrophins– Neurotrophins are a family of proteins that promote the survival, development, and function of neurons.
Homeostatic– The process by which an organism tends to maintain stability while adjusting to the best conditions for survival is termed homeostasis.
Wnt– The Wnt signaling pathway consists of a group of signal transduction pathways that begin with proteins that transmit signals to cells via cell surface receptors.
Sonic hedgehog (Shh)– Sonic hedgehog is a protein that is encoded by the SHH gene. Different types of animals use this signaling molecule to regulate embryonic morphogenesis.
Notch signaling pathway– Notch is a highly conserved signaling pathway that is found in most animals.
Calbindin– it function is in mediating calcium absorption.
References
- Shoji M. (2004). Biomarkers of Alzheimer’s disease. Clinical Neurology, 44(11), 771-774. doi:10.1373/jalm.2019.030080
- Ferreira A. (2012). Calpain Dysregulation in Alzheimer ’ s Disease. International Scholarly Research Notices, 2012 (2012). doi:10.5402/2012/728571
- Malve HO.(2017). Management of Alzheimer’s disease: Role of existing therapies, traditional medicines and new treatment targets. Indian J Pharm Sci, 79(1),2-15. doi:10.4172/pharmaceutical-sciences.1000195
- Sosa-Ortiz AL, Acosta-Castillo I, Prince MJ.(2012). Epidemiology of Dementias and Alzheimer’s Disease. Arch Med Res, 43(8), 600-608. doi:10.1016/j.arcmed.2012.11.003
- World Health Organization.(2009). Neurological Disorders: Public Health Challenges. https://www.who.int/publications/i/item/9789241563369
- Imbimbo BP, Lombard J, Pomara N.(2005). Pathophysiology of Alzheimer’s disease. Neuroimaging Clin N Am, 15(4), 727-753. doi:10.1016/j.nic.2005.09.009
- Hsu D, Marshall GA.(2016). Primary and Secondary Prevention Trials in Alzheimer Disease: Looking Back, Moving Forward. Curr Alzheimer Res, 14(4), 426-440. doi:10.2174/1567205013666160930112125
- Braak H, Braak E. (1991). Morphologische veranderungen der menschlichen endhirnrinde bei demenz. Journal Fur Hirnforschung, 32(3), 277-282.
- Altman J, Das G D.(1965). Autoradiographic and Histoloaical Evidence of. Journal of Comparative Neurology, 124(3), 319-335.
- Gage FH.(2000). Mammalian neural stem cells. Science, 287(5457), 1433-1438. doi:10.1126/science.287.5457.1433.
- Scopa C, Marrocco F, Latina V, et al.(2020). Impaired adult neurogenesis is an early event in Alzheimer’s disease neurodegeneration, mediated by intracellular Aβ oligomers. Cell Death Differ, 27(3), 934-948. doi:10.1038/s41418-019-0409-3
- Kempermann G, Gage FH, Aigner L, et al.(2018). Human Adult Neurogenesis: Evidence and Remaining Questions. Cell Stem Cell, 23(1), 25-30. doi:10.1016/j.stem.2018.04.004
- Tobin MK, Musaraca K, Disouky A, et al.(2019). Human Hippocampal Neurogenesis Persists in Aged Adults and Alzheimer’s Disease Patients. Cell Stem Cell, 24(6), 974-982.e3. doi:10.1016/j.stem.2019.05.003
- Atri A.(2019). The Alzheimer’s Disease Clinical Spectrum: Diagnosis and Management. Med Clin North Am, 103(2), 263-293. doi:10.1016/j.mcna.2018.10.009
- NHS.(2021). Alzheimer’s disease – Causes. https://www.nhs.uk/conditions/alzheimersdisease/causes/
- Tackenberg C, Kulic L, Nitsch RM.(2020). Familial Alzheimer’s disease mutations at position 22 of the amyloid β-peptide sequence differentially affect synaptic loss, tau phosphorylation and neuronal cell death in an ex vivo system. PLoS One, 15(9). doi:10.1371/journal.pone.0239584
- Hashimoto M, Rockenstein E, Crews L, Masliah E.(2003). Role of Protein Aggregation in Mitochondrial Dysfunction and Neurodegeneration in Alzheimer’s and Parkinson’s Diseases. NeuroMolecular Med, 4(1-2), 21-35. doi:10.1385/NMM:4:1-2:21
- Wenk GL.(2006). Neuropathologic changes in Alzheimer’s disease: Potential targets for treatment. J Clin Psychiatry, 67(3), 3-7.
- Deture MA, Dickson DW.(2019). The neuropathological diagnosis of Alzheimer’s disease. Mol Neurodegener, 14(1). doi:10.1186/s13024-019-0333-5
- Desikan RS, Cabral HJ, Hess CP, et al.(2009). Automated MRI measures identify individuals with mild cognitive impairment and Alzheimers disease. Brain, 132(8), 2048-2057. doi:10.1093/brain/awp123
- Webers A, Heneka MT, Gleeson PA.(2020). The role of innate immune responses and neuroinflammation in amyloid accumulation and progression of Alzheimer ’ s disease. Immunology and cell biology, 98(1), 28-41. doi:10.1111/imcb.12301
- Turner PR, O’Connor K, Tate WP, Abraham WC.(2003). Roles of amyloid precursor protein and its fragments in regulating neural activity, plasticity and memory. Prog Neurobiol, 70(1), 1-32. doi:10.1016/S0301-0082(03)00089-3
- Priller C, Bauer T, Mitteregger G, Krebs B, Kretzschmar HA, Herms J.(2006). Synapse formation and function is modulated by the amyloid precursor protein. J Neurosci, 26(27), 7212-7221. doi:10.1523/JNEUROSCI.1450-06.2006
- Swerdlow RH.(2007). Pathogenesis of Alzheimer’s disease. Clin Interv Aging, 2(3), 347-359.
- Michaelis ML, Dobrowsky RT, Li G.(2002). Tau neurofibrillary pathology and microtubule stability. J Mol Neurosci, 19(3), 289-293. doi:10.1385/JMN:19:3:289
- Terwel D, Dewachter I, Van Leuven F.(2002). Axonal transport, tau protein, and neurodegeneration in Alzheimer’s disease. NeuroMolecular Med, 2(2), 151-165. doi:10.1385/NMM:2:2:151
- GREIG NH.(2004). New Therapeutic Strategies and Drug Candidates for Neurodegenerative Diseases: p53 and TNF- Inhibitors, and GLP-1 Receptor Agonists. Ann N Y Acad Sci, 1035(1), 290-315. doi:10.1196/annals.1332.018
- Tapia-Arancibia L, Aliaga E, Silhol M, Arancibia S.(2008). New insights into brain BDNF function in normal aging and Alzheimer disease. Brain Res Rev, 59(1), 201-220. doi:10.1016/j.brainresrev.2008.07.007
- Schindowski K, Belarbi K, Buée L.(2008). Neurotrophic factors in Alzheimer’s disease: Role of axonal transport. Genes, Brain and Behavior, 7(29) , 43-56.doi:10.1111/j.1601-183X.2007.00378.x
- Trumpler, M. (1994). Cajal’s Degeneration and Regeneration of the Nervous System by Santiago Ramon y Cajal; Raoul M. May; Javier DeFelipe; Edward G. Jones. Isis, 85(3), pp.543-544.
- Sidman RL, Miale IL, Feder N.(1959). Cell proliferation and migration in the primitive ependymal zone : an autoradiographic study of histogenesis in the nervous system. Exp Neurol, 1(4), 322-333. doi:10.1016/0014-4886(59)90024-X
- Altman J.(1966). Autoradiographic and histological studies of postnatal neurogenesis. I. A longitudinal investigation of the kinetics, migration and transformation of cells incoorporating tritiated thymidine in neonate rats, with special reference to postnatal neurogenesi. J Comp Neurol, 126(3), 337-389. doi:10.1002/cne.901260302
- Altman J.(1969). Autoradiographic and histological studies of postnatal neurogenesis. IV. Cell proliferation and migration in the anterior forebrain, with special reference to persisting neurogenesis in the olfactory bulb. J Comp Neurol, 137(4), 433-457. doi:10.1002/cne.901370404
- Gratzner, H. G. (1982). Monoclonal Antibody to 5-Bromo- and 5-Iododeoxyuridine : A New Reagent for Detection of DNA Replication Placental Mononuclear Phagocytes as a Source of Interleukin-1. Science, 218(4571), 474-475.
- Reynolds BA, Weiss S.(1992). Generation of Neurons and Astrocytes from Isolated cells of adult mammalin central nervous system. science, 255(5052)1707-1710.
doi:10.1126/science.1553558
- Kukekov, V. G., Laywell, E. D., Suslov, O., Davies, K., Scheffler, B., Thomas, L. B.,… & Steindler, D. A. (1999). Multipotent stem/progenitor cells with similar properties arise from two neurogenic regions of adult human brain. Experimental neurology, 156(2), 333-344.
- Kempermann, G., & Gage, F. H. (1999). New nerve cells for the adult brain. Scientific American, 280(5), 48-53.
- Belluzzi O, Benedusi M, Ackman J, LoTurco JJ.(2003). Electrophysiological Differentiation of New Neurons in the Olfactory Bulb. J Neurosci, 23(32), 10411-10418. doi:10.1523/jneurosci.23-32-10411.2003
- Praag V, Af S, Br C, N T, Td P, Fh G.(2002). Functional neurogenesis in the adult hippocampus. Nature, 415(6875), 1030-1034.
- Boldrini M, Fulmore CA, Tartt AN, et al.(2018). Human Hippocampal Neurogenesis Persists throughout Aging. Cell Stem Cell, 22(4), 589-599.e5. doi:10.1016/j.stem.2018.03.015
- Schinder AF, Gage FH.(2004). A hypothesis about the role of adult neurogenesis in hippocampal function. Physiology, 19(5), 253-261. doi:10.1152/physiol.00012.2004
- Mu Y, Gage FH.(2011). Adult hippocampal neurogenesis and its role in Alzheimer’s disease. Mol Neurodegener, 6(1), 1-9. doi:10.1186/1750-1326-6-85
- Mu Y, Lee SW, Gage FH.(2010). Signaling in adult neurogenesis. Curr Opin Neurobiol, 20(4), 416-423. doi:10.1016/j.conb.2010.04.010
- Ming GL, Song H.(2005). Adult neurogenesis in the mammalian central nervous system. Annu. Rev. Neurosci., 28,(1) 223-250. doi:10.1146/annurev.neuro.28.051804.101459
- Obernier K, Cebrian-Silla A, Thomson M, et al.(2018). Adult Neurogenesis Is Sustained by Symmetric Self-Renewal and Differentiation. Cell Stem Cell, 22(2), 221-234.e8. doi:10.1016/j.stem.2018.01.003
- Curtis MA, Kam M, Nannmark U, et al.(2007). Human neuroblasts migrate to the olfactory bulb via a lateral ventricular extension. Science, 315(5816), 1243-1249. doi:10.1126/science.1136281
- Carleton A, Petreanu LT, Lansford R, Alvarez-Buylla A, Lledo PM.(2003). Becoming a new neuron in the adult olfactory bulb. Nat Neurosci, 6(5), 507-518. doi:10.1038/nn1048
- Marín-Burgin A, Schinder AF.(2012). Requirement of adult-born neurons for hippocampus-dependent learning. Behav Brain, 227(2), 391-399. doi:10.1016/j.bbr.2011.07.001
- Aimone JB, Li Y, Lee SW, Clemenson GD, Deng W, Gage FH.(2014). Regulation and function of adult neurogenesis: from genes to cognition. Physiol Rev, 94(4), 991-1026. doi:10.1152/physrev.00004.2014
- Sahay A, Scobie KN, Hill AS, et al.(2011). Increasing adult hippocampal neurogenesis is sufficient to improve pattern separation. Nature, 472(7344), 466-470. doi:10.1038/nature09817
- Kumar D, Koyanagi I, Carrier-Ruiz A, et al.(2020). Sparse Activity of Hippocampal Adult-Born Neurons during REM Sleep Is Necessary for Memory Consolidation. Neuron, 107(3), 552-565.e10. doi:10.1016/j.neuron.2020.05.008
- Cameron HA, Mckay RDG.(2001). Adult neurogenesis produces a large pool of new granule cells in the dentate gyrus. J Comp Neurol, 435(4), 406-417. doi:10.1002/cne.1040
- Bear, M., Connors, B., & Paradiso, M. A. (2020). Neuroscience: Exploring the Brain, Enhanced Edition: Exploring the Brain, Enhanced Edition (4th ed.). Jones & Bartlett Learning
- Faigle R, Song H.(2013). Signaling mechanisms regulating adult neural stem cells and neurogenesis. Biochim Biophys Acta – Gen Subj, 1830(2), 2435-2448. doi:10.1016/j.bbagen.2012.09.002
- Anacker C, Hen R.(2017). Adult hippocampal neurogenesis and cognitive flexibilitylinking memory and mood. Nat Rev Neurosci, 18(6), 335-346. doi:10.1038/nrn.2017.45
- Trinchero MF, Buttner KA, Sulkes Cuevas JN, et al.(2017). High Plasticity of New Granule Cells in the Aging Hippocampus. Cell Rep, 21(5), 1129-1139. doi:10.1016/j.celrep.2017.09.064
- Kempermann G.(2015). Activity dependency and aging in the regulation of adult neurogenesis. Cold Spring Harb Perspect Biol, 7(11), 1-19. doi:10.1101/cshperspect.a018929
- Arber C, Lovejoy C, Harris L, et al.(2021). Familial Alzheimer’s Disease Mutations in PSEN1 Lead to Premature Human Stem Cell Neurogenesis. Cell Rep, 34(2), 108615. doi:10.1016/j.celrep.2020.108615
- Moreno-Jiménez EP, Flor-García M, Terreros-Roncal J, et al.(2019). Adult hippocampal neurogenesis is abundant in neurologically healthy subjects and drops sharply in patients with Alzheimer’s disease. Nat Med, 25(4), 554-560. doi:10.1038/s41591-019-0375-9
- Lazarov O, Marr RA.(2010). Neurogenesis and Alzheimer’s disease: At the crossroads. Exp Neurol, 223(2), 267-281. doi:10.1016/j.expneurol.2009.08.009
- Scardigli R, Capelli P, Vignone D, et al.(2014). Neutralization of nerve growth factor impairs proliferation and differentiation of adult neural progenitors in the subventricular zone. Stem Cells, 32(9), 2516-2528. doi:10.1002/stem.1744
- Shelanski ML.(2007). Increased neurogenesis in young transgenic mice overexpressing human APP, Journal of Alzheimer’s Disease, 12(3), 229-240.
- Demars M, Hu YS, Gadadhar A, Lazarov O.(2010). Impaired neurogenesis is an early event in the etiology of familial Alzheimer’s disease in transgenic mice. J Neurosci Res, 88(10), 2103-2117. doi:10.1002/jnr.22387
- Hollands C, Tobin MK, Hsu M, et al.(2017). Depletion of adult neurogenesis exacerbates cognitive deficits in Alzheimer’s disease by compromising hippocampal inhibition. Mol Neurodegener, 12(1), 1-13. doi:10.1186/s13024-017-0207-7
- Wang, Q., Matsumoto, Y., Shindo, T., Miyake, K., Shindo, A., Kawanishi, M., Nagao, S. (2006). Neural stem cells transplantation in cortex in a mouse model of Alzheimer’s disease. The Journal of Medical Investigation, 53(1-2), 61-69. doi: 10.2152/jmi.53.61
- Papadimitriou C, Celikkaya H, Cosacak MI, et al.(2018). 3D Culture Method for Alzheimer’s Disease Modeling Reveals Interleukin-4 Rescues Aβ42-Induced Loss of Human Neural Stem Cell Plasticity. Dev Cell, 46(1), 85-101.e8. doi:10.1016/j.devcel.2018.06.005
- Trejo JL, LLorens-Martín M V., Torres-Alemán I.(2008). The effects of exercise on spatial learning and anxiety-like behavior are mediated by an IGF-I-dependent mechanism related to hippocampal neurogenesis. Mol Cell Neurosci, 37(2), 402-411. doi:10.1016/j.mcn.2007.10.016
- Choi SH, Bylykbashi E, Chatila ZK, et al.(2018). Combined adult neurogenesis and BDNF mimic exercise effects on cognition in an Alzheimer’s mouse model. Science, 361(6406). doi:10.1126/science.aan8821
Phytochemicals – a New Pipeline for Anticancer Drug Development
Foram Patel*, Denni Mammen, Elizabeth Robin and Darshee Baxi
School of Science, Navrachana University, Vasna-Bhayli Road, Vadodara-391 410, Gujarat, India
Received: 26 August 2021 Revised: 12 January 2022 Accepted: 20 January 2022 Published: 3 March 2022
*Corresponding Author: foramp@nuv.ac.in
Abstract
In spite of advance interventions and novel strategies, cancer is still one of the major causes of mortality worldwide. The constant increase in cancer patients, failure of conventional chemotherapeutics due to toxicity clearly demands an alternative approach. In past decades, scientific community is engrossed in the discovery of new agents from natural sources. The phytochemicals have recently gained the attention because of their potential to modulate cascades of molecular mechanisms that governs the tumor growth and progression. The protective effects of phytochemicals have gained attention due to less side effects and greater safety index. The present review summarizes the perspective of the scientific evidences as well as their potential druggability. The approach aimed to examine the current status of phytochemical compounds currently used to treat cancer and their potential at a preclinical and clinical level. It also addresses current challenges that lie ahead for their use. The methodology adopted for this review was comparative analysis of the papers published in the areas of antitumor plant-based products or plant compounds or dietary phytochemicals, anticancer phytochemicals or anticancer herbs. The analysis involved papers published from 2010 to 2020 for the recent understanding.
Keywords
Cancer, Secondary metabolites, Phytochemicals, Phyto-agents
Introduction
Cancer is a significant public health problem worldwide and is the second leading cause of death. Cancer is a large group of diseases that begin in any organ or tissue of the body when abnormal cells grow wildly, go past their usual limits to attack connecting parts of the body and/or spread to other organs. The latter process is called metastasizing and is a significant reason of death from malignancy. A neoplasm and malignant tumors are commonly considered for cancer. According to the World Health Organization (WHO), it is estimated that globally 9.6 million deaths occur due to cancer and its complications. Lung, colorectal, prostate, liver and liver cancer are well known types of cancer in men, while breast, cervical, thyroid, lung and colorectal cancer are well known among the women. The economic burden continues to grow due to lack of proper health management systems. The current treatment options available for treatments are chemotherapy and radiation therapy while sometimes surgical removal is also opted. The major disadvantage due to the chemotherapeutics is relapse of cancer, drug resistance and toxicity to the non-targeted tissues. These problems resist the use of the currently available chemotherapeutics drugs as it ultimately impairs the quality of life. Therefore, there is urgent need to search new lead anticancer agents with better potency and lesser complications. There are many scientific evidences available which shows that natural compounds are good sources for the development of new remedies for cancer. 1 There has been investigation that floral kingdom consists of approximately 250000 plant species and nearby 10% have been researched for the cancer treatment.2 The plant derived analogues are present in different plant and have several pharmacological functions. There is wide research gap and investigation is needed to identify the molecules involved in cell signaling network and better assessment is needed in understanding their mechanism of action. Another research need is dosage concentration and frequency of intake at human trials. However, an important research need is testing in vitro dosage which are unachievable in human trials.3-6 Phytochemicals serve as the promising candidate for the treatment of the cancer. For example, currently Taxol analogues, Vinca alkaloids and podophyllotoxins analogues has been used for the treatment. This paper aims to review the available biological actions of phytoconstituent in relation to cancer treatments. It also attempts to investigate the current concerns of chemotherapeutics being used in practice.
Challenges and introspection into the current cancer treatment regimen:
There are three major aspects for clinical trials with phytochemicals: 1) adjuvant for chemo and radiotherapy. 2) reduction of chronic side effects of chemotherapy, and 3) unwanted interaction with chemo and radiotherapy. The array of compounds like berberine, curcumin, epigallocatechin, quercetin, resveratrol and sulphorafane are currently in clinical trials on various cancers. The major classes of plant-based anticancer compounds clinically used include epipodophyllotoxin, camptothecin derivatives, vinca alkaloids, and taxane diterpenoids. However, there still remains the key concern of poor solubility, poor penetration in target cells, limited therapeutic potential and toxicity. In this regard, prodrugs, combination therapy with conventional chemotherapeutics, synthetic metal analogues and nano-formulations could be targeted to enhance the bioactivity. These major limitations can be overcome with the advent of nanotechnology and micro-encapsulation of phytochemical for targeted delivery, longer circulation period in the bloodstream and lesser side effects over free compounds. The combinational approach with mixture/single of phytochemicals and chemotherapeutic drugs induce synergistic effect and may be efficient at the lower dose compared to an individual drug which increases toxicity in normal cells. The use of cholesterol/sphingomyelin liposomal vincristine (Mariqbo®) for the treatment of acute lymphoblastic leukemia was approved by Food and Drug Administration (FDA).7 Paclitaxel is considered to be a magic pill in chemotherapeutic not only used as a single drug but combined with other anticancer drugs. The hormone-refractory metastatic breast and prostate cancer is treated with Cabazitaxel (Jevtana®) approved by FDA.8 These modifications in delivery system have increased accumulation of drugs in target cells and improved their cytotoxicity. When docetaxel combined with curcumin administered in mice having breast cancer showed reversal in drug resistance.9 Recently it has been reported that the gingerol increased the sensitivity of cisplatin in gastric cancer and doxorubicin in liver cancer.10-11
An overview of Phytochemicals
Phytochemicals, or ‘plant chemicals’, are bioactive non-nutrient plant compounds that has ability to interact with at least one component of a living tissue introducing enormous scope of credible effects. They shield plants from destructive microorganisms and also from ultraviolet (UV) irradiation and extreme temperatures. Additionally, birds and insects are attracted to promote seed dispersal, pollination and germination. Phytochemicals are responsible for colours to plants and range of flavours both pleasant and unpleasant when consumed. They are explicit to specific plants and parts of plants, and that they usually synthesis is increased during stressful events. Phytochemicals additionally provide health benefits when plant or its part are devoured. They consist of biomolecules essential for well-being (e.g., proteins, carbohydrates, vitamins, and minerals) and other supplements (e.g., phenolic acids, flavonoids, and other phenolics).12 Phytochemicals are classified as primary metabolites and secondary metabolites. Primary metabolites comprise of the common sugars, amino acids, proteins, nitrogenous bases pyrimidines and purines, chlorophylls etc. Secondary metabolites include alkaloids, flavonoids, steroids, terpenes, lignans, saponins, phenolics and glucosides. The detailed classification is represented in Fig 1.
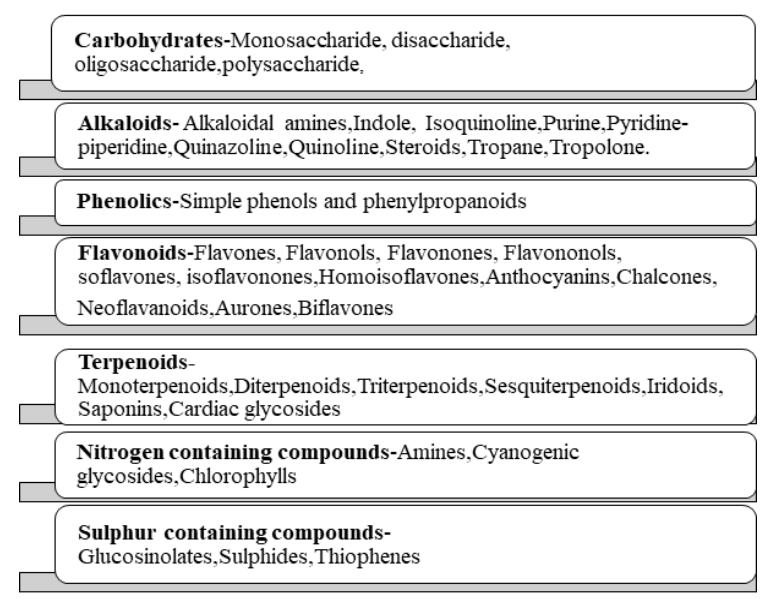
Figure 1: Classification of Phytochemicals
Role of Phytochemicals in cancer treatment
Plants are indispensable sources for anticancer drug development. Natural dietary phytochemicals are broadly utilized in in vitro, in vivo, and preclinical cancer prevention and treatment studies. In number of clinical trials have shown various degrees of success. Since ancient times plants and their formulations are used for its probable effects. In any case, therapy of plant-based compounds for the treatment of cancer can be followed back to 1950s. Some of the very first anticancer agents derived from plants are vinca alkaloids such as vinblastine, vincristine, and cytotoxic podophyllotoxins. Modern drug development program based on Ayurveda and has acquired acknowledgement in present healthcare settings. Plant derived natural products are nontoxic to normal cells and also better endured henceforth they have acquired consideration for modern drug discovery. Alkaloids, flavonoids, phenolics, tannins, glycosides, gums, resins and oils and their derivatives present in root, stem, bark leaf, and flower perform many pharmacological functions in human systems. Vinblastine, vincristine, taxol, elliptinium, etoposide, colchicinamide, 10-hydroxycamptothecin, curcumol, gossypol, ipomeanol, lycobetaine, tetrandrine, homoharringtonine, monocrotaline, curdione, and indirubin are astounding phyto molecules in this scenario.13 The extraordinary potential of plant-based compounds for the treatment and counteraction of cancer is ascribed to their safety, low cost, and oral bioavailability. However, a couple of plant-based compounds induce some side effects.It also has been elucidated that phytochemical can modulate key cellular signalling pathways from initiation to progression by targeting different stages. The exposure of carcinogen at cellular, genetic and epigenetic levels triggers the multistep carcinogenesis process. The initial uptake of carcinogenic agent and its assimilation in tissues where its activation and detoxification occur, interaction with the DNA, results in genetic damage and transformation into neoplastic cells.14 The tumour promotion on other hand is relatively expanded proliferation of cancerous cells regulated by mediators of cell signalling pathways like receptors, regulatory proteins, kinases and cyclins. The tumour promotion on other hand is relatively expanded proliferation of cancerous cells regulated by mediators of cell signalling pathways like receptors, regulatory proteins, kinases and cyclins. The final stage is progression of neoplastic transformation characterized by metastatic potential and migration to the distant location in the body. Thus this bidirectional communication between cells and their secondary sites serve as one of the promising target for the action of phytocompounds.15 For instance, to name a few dietary phytochemicals like quercetin from onion, apple, lemon, Epigallocatechinfrom green tea, genistein from soya bean, caffeic acid from coffee, resveratrol from red grapes, berries, Indol-3-carbinol from cruciferous vegetables have been proved in extensive range of anti-proliferation, cell cycle blockage, DNA repair alterations, apoptosis induction, free radical scavenging, anti-inflammation, activation of tumour suppressor genes, suppression of oncogenes, regulation of growth factors and hormones and inhibition of invasion, angiogenesis and metastasis.16 The molecular connection between the phytochemicals and regulatory check points of cancer progression is illustrated in the fig.2. Several crude phytochemicals which are obtained from medicinal plants and has multifactorial effects on the many types of cancer are as depicted below.
Important phytochemicals and their effect on types of cancer:
1. Andrographolide
Species: Andrographis paniculata
Andrographolide is one of the diterpene lactone found in the Andrographis paniculata which is reported to exert the anti-proliferative activity on various human cancer cells.17The anti-cancer activity is modulated through cell-cycle arrest by induction of p27 accompanied with decrease in cyclin dependent kinase 4 (CDK4) expression. Further, the immunomodulatory activity was reported by increase in cytokine levels and enhanced natural killer cell activity. These demonstrate andrographolide as an opportunistic pharmacophore with potent anticancer/immunomodulatory activity.18
2. Berberine
Species: Berberis vulgaris
Berberine is a benzylisoquinoline alkaloid present in the roots, rhizomes, stem and bark of B. vulgaris. Berberine has multifaceted role like cell cycle arrest, autophagy, inhibition of cell invasion and metastasis, regulation in tumour microenvironment and immunomodulatory activity.19 Also it has been demonstrated in vivo levels of catalase and glutathione peroxidase enzymes increased which proves berberine as a potent anti-oxidant.20
3. Crocetin
Species: Crocus sativus
Crocetin is a diterpenoid and natural carotenoid found in crocus flower. There are reports which shows that crocetin have significant effect on Breast, Cervical, Colorectal, Leukemia, Liver, Lung, Pancreas, Skin.21 Crocetin exert anti-proliferative, anti-apoptotic, and decrease the activity of RNA and DNA polymerase. It also decreases the lipid peroxidation with concomitant increase in GST, catalase and superoxide dismutase.22-23
4. Curcumin
Species: Curcuma longa
Curcumin is polyphenol extracted from rhizome of Curcuma longa also known as turmeric. Several studies demonstrated curcumin’s anti-cancer activity on leukemia, breast, gastric, pancreas, colorectal, prostate, cervical, liver and lung.24 The curcumin mainly exerts its activity by prompting apoptosis and restraining proliferation and invasion of tumors by suppressing cellular signalling pathways like including Wnt/β-catenin, PI3K/Akt, JAK/STAT, MAPK, p53 and NF-ĸB.25
5. Aloe emodin
Species: Aloe barbadensis
Aloe emodin is dihydroxyanthraquinone present in sap of aloe vera. It exhibits an array of deleterious effect on cancers like ovary, colorectal carcinoma, gastric carcinoma, liver, glioma, leukemia, breast, small cell lung cancer including reduction in cell viability, induction in cell cycle arrest, apoptosis, cell cycle arrest through downregulation of cyclin dependent and independent kinase. Additionally, emodin related compounds also served as adjuvants with chemotherapy in treatment of certain cancers.26-27
6. Magniferin
Species: Mangifera indica
Magniferin is xanthoid present in the peel, stalks, leaves, barks, kernel, and seed of mango fruit. Magniferin showed anti-proliferative effect on breast, prostate, pancreas, lung, colon, leukemia and cervical cancer.28 Further it causes cell cycle arrest at G2/M phase accompanied with the inhibition of expression levels of proteins like ATR, Chk1, Wee1, Akt, and Erk1/2. It also relieves the oxidative stress, suppress metastatic potential, decrease the expression of MMP-7 and 9, and inhibit b-actin pathway by reversing epithelial-mesenchymal transition (EMT).29
7. Plumbagin
Species: Plumbago zeylanica
Plumbagin is a naphthoquinone isolated from roots of Plumbago zeylanica. There are reports which demonstrated the role of Plumbagin against gastric, breast, melanoma, promyelocytic leukemia cancer. It has been reported that plumbagin suppress malignant activity of tumour cells through cascade of mechanism such as the inhibition of growth, invasion, metastasis and anti-angiogenesis.30 Flow cytometric analysis of human cancer cell lines revealed that Plumbagin arrest cell cycle at G1 phase with concomitant inhibition of cyclin D1, cyclin E, and upregulation of p53.31
8. Piperine
Species: Piper longum
Piperine is an alkaloid transcendently found in the fruits and roots of Piper longum. Black pepper has been exploited as king of spices in Indian system of medicine for various ailments.32 Piperine not only exhibits anti-proliferative activity but also regulates the fundamental proteins involved in the cancer progression. Piperine inhibits cell proliferation via cell cycle arrest, triggers reactive oxygen species production which in turn activates intrinsic and extrinsic apoptosis pathways. Piperine at the molecular level block the Akt phosphorylation leading to inhibition of angiogenesis and vascular endothelial growth factor (VEGF).33
9. Epigallocatechin
Species: Camellia sinensis
Epigallocatechin (type of catechin) is a polyphenol extracted from tea plant. The epigallocatechin mediates the anti-cancer activity via inhibition of nuclear factors associated with the inhibition of migration, invasion and angiogenesis. It has dual function of anti-oxidant and pro-oxidant potential which modulates the production of reactive oxygen species leading to epigenetic modification, regulation of histone acetylation and upregulation of apoptosis.34-35
10. Resveratrol
Species: Vitis vinifera
Resveratrol is trans-stilbenoid polyphenol present predominantly in grapes but also found in mulberries, blueberries, cranberries and peanut. Resveratrol activates apoptotic pathways which includes increase in levels of Bax and decrease in levels of Bcl-2 and cyclin D1. In addition to this it regulates cell differentiation, DNA repair, cell cycle arrest, autophagy, angiogenesis and metastasis.36 It also modulates the balance of cyclins as well as cyclindependent kinases (CDKs) which inhibits cell cycle at G0-G1 phase. It activates the STAT3 which promotes the proliferation, survival, invasion, angiogenesis, and metastasis of tumour cells.37
Phytochemical |
Type of cancer |
Biological action |
Andrographolide38-39 |
Breast, Liver, Lung,Ovarian, Cervical |
suppression of heat shock protein 90, cyclins and cyclin-dependent kinases, metalloproteinase and growth factors, and the induction of tumour suppressor proteins p53 and p21. |
Berberine40-42 |
Breast, Liver, Lung, Ovarian, Cervical, Prostate, Colorectal |
Caspase activation; ROS production, Cytochrome c release;Bcl-2/Bcl-xL decrease, COX-2 downregulation |
Crocetin43-45 |
Breast, Cervical, Colorectal, Leukemia, Liver, Lung, Pancreas, Skin. |
suppression of Bcl-2 and up-regulation of Bax expression, |
Curcumin46-48 |
Blood, Breast, Gastric, Pancreas, Colorectal, Prostate, Cervical, Liver, Lung, Skin |
interfere with multiple cellular signalling cascades including Wnt/β-catenin signalling, phosphoinositide 3-kinase (PI3K)/protein kinase B (Akt) pathway, Janus kinase (JAK)/signal transducer and activator of transcription (STAT) signalling pathway, mitogen-activated protein kinase (MAPK) pathway, p53 signalling and nuclear factor-ĸB (NF-ĸB) pathway |
Aloe emodin49-50 |
Ovary, Colorectal carcinoma, Gastric carcinoma, Liver, Glioma, Blood, Breast |
cell cycle arrest through downregulation of cyclin dependent and independent kinase and suppression of Bcl-2 and upregulation of Bax expression |
Magniferin51 |
Breast, Liver, Lung, Blood, Prostate, Brain, Gastric, Kidney |
down-regulation of inflammation, cell cycle arrest, reduction of proliferation/metastasis, promotion of apoptosis in malignant cells and protection against oxidative stress and DNA damage. |
Plumbagin52-54 |
Breast, Liver, Lung,Blood, Prostate, Brain, Gastric, Kidney |
Cell cycle arrest, DNA damage, apoptosis, and suppression of telomere and telomerase activity, inhibition of proteasome, Inhibition of COX-2 and STAT3 signalling pathway |
Piperine55-56 |
Breast ,Lung, Prostate, cervical, Ovary, colorectal |
induction cell cycle arrest, increased cell apoptosis, disruption of redox homeostasis, inhibition of angiogenesis, modulation in stress, and autophagy, influence on the Wnt/β-catenin and inhibition of PI-3K/Akt signalling pathways |
Epigallocatechin57-58 |
Breast, Lung, Pancreatic, Ovary, Oral, Prostate, Blood, Colorectal, |
induction of apoptosis, inhibiting NF-ĸB activation and downregulate the key genes associated with angiogenesis, tumour metastasis and survival. |
Resveratrol59-60 |
Breast, Colon, Liver, Lung, Prostate, Blood |
induction cell cycle arrest, increased cell apoptosis, inhibition of COX-2 and STAT3 signalling pathway |
Table 1: Summary of phytochemicals used in cancer treatment.
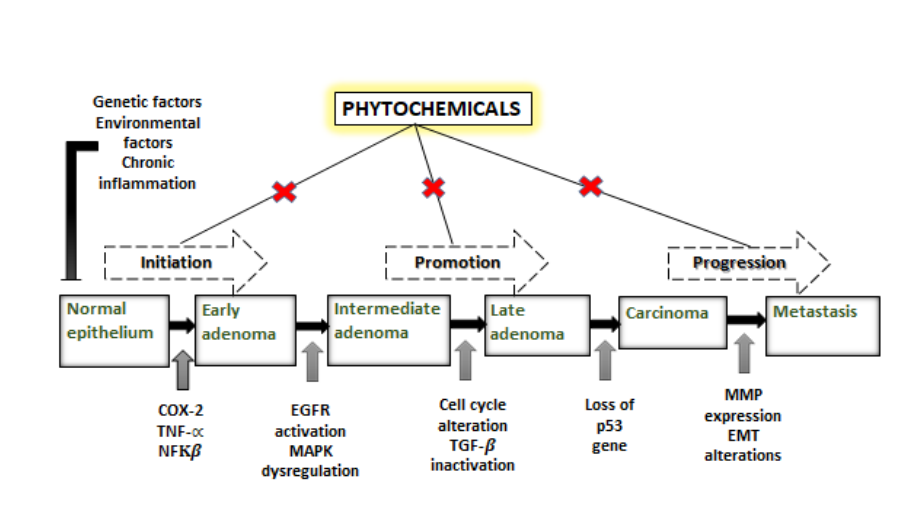
Figure 2: Phytochemicals alterate the key signalling pathways of cancer from initiation to progression.
Conclusion and Future perspective:
The high biodegradability and biocompatibility of phytocompounds have open the new avenues in cancer therapy. The phytochemicals can be categorized as signalling molecules and imposition of serving as phyto-chemotherapeutics is still needed to be investigated. The phytocompounds could be combined with the conventional therapies to enhance the potency and prevent tumour recurrence after achieving a successful treatment. The pleotropic properties of Phyto agents can be considered as pioneering adjuvant line of action that can be in combination with chemotherapeutics. In conventional approach of research, efficacy of phytochemicals is evaluated in vitro and then in vivo followed by mechanistic studies. This screening only suffices the cytotoxic effect on cancer cells. To overcome these limitations, the approach should be developed to predict the pharmacological activities by using OMICS analyses. Although there are many known phytochemicals having cytotoxic effects, but the exact molecular/cellular targets and systematic mechanisms for many of them is questionable. With the advancement of bioinformatics, the in-silico approaches to study the pharmacokinetics properties of molecules should be opted. So due to large scale use of traditional healthcare products and their demand by humans, it is very essential to scrutinize different formulations for anticancer therapy in vitro as well as in vivo.
Glossary:
- Neoplasm– an abnormal growth of tissue caused due to rapid cell division. It is also known as tumour.
- Malignant tumors– cancerous tumor.
- Chemotherapeutics– drugs for treatment of cancer.
- Adjuvant– substance which help or aid to increase the potency of the drug.
- Alkaloid– a class of naturally occurring heterocyclic nitrogen bases in plants having pharmacological effects.
- Flavonoids– a class of complex polyphenolic compounds naturally present in plants.
- Terpenoids– also known as isoprenoids.
- Tannins– water soluble polyphenol naturally present in plants.
- Apoptosis– It’s a programmed cell death.
- Metastasis– It’s a process through which cancer cells spreads to other body parts.
- Adenoma– structurally unorganized neoplasm consisting of fibrous, glandular and fat tissues.
- Carcinoma– most common type of malignancy starts at inner or outer epithelium of the organs.
References:
- Solowey, E., Lichtenstein, M., Sallon, S., Paavilainen, H., Solowey, E., & LorberboumGalski, H. (2014). Evaluating medicinal plants for anticancer activity. The Scientific World Journal, 2014, Article ID 721402 ,12-24. DOI: https://doi.org/10.1155/2014/721402
- Singh, S., Jarial, R., & Kanwar, S. S. (2013). Therapeutic effect of herbal medicines on obesity: herbal pancreatic lipase inhibitors. Wudpecker J Med Plants, 2(1), 53-65.
- Yadav, V. R., Prasad, S., Sung, B., & Aggarwal, B. B. (2011). The role of chalcones in suppression of NF-κB-mediated inflammation and cancer. International Immunopharmacology, 11(3), 295-309.
- Tanaka, T., Shnimizu, M., & Moriwaki, H. (2012). Cancer chemoprevention by carotenoids. Molecules, 17(3), 3202-3242.
- Zhuang, S. R., Chen, S. L., Tsai, J. H., Huang, C. C., Wu, T. C., Liu, W. S., & Wang, C. K. (2009). Effect of citronellol and the Chinese medical herb complex on cellular immunity of cancer patients receiving chemotherapy/radiotherapy. Phytotherapy Research: An International Journal Devoted to Pharmacological and Toxicological Evaluation of Natural Product Derivatives, 23(6), 785-790.
- Sharma, H., Parihar, L., & Parihar, P. (2011). Review on cancer and anticancerous properties of some medicinal plants. Journal of Medicinal Plants Research, 5(10), 1818- 1835.
- Wang, X., Song, Y., Su, Y., Tian, Q., Li, B., Quan, J., & Deng, Y. (2016). Are PEGylated liposomes better than conventional liposomes? A special case for vincristine. Drug Delivery, 23(4), 1092-1100.
- de Morrée, E., van Soest, R., Aghai, A., de Ridder, C., de Bruijn, P., Ghobadi Moghaddam‐Helmantel, I. & van Weerden, W. (2016). Understanding taxanes in prostate cancer; importance of intratumoral drug accumulation. The Prostate, 76(10), 927-936.
- Aung, T. N., Qu, Z., Kortschak, R. D., & Adelson, D. L. (2017). Understanding the effectiveness of natural compound mixtures in cancer through their molecular mode of action. International Journal of Molecular Sciences, 18(3), 656.
- Luo, Y., Zha, L., Luo, L., Chen, X., Zhang, Q., Gao, C., & Qiao, T. (2019). [6] ‐Gingerol enhances the cisplatin sensitivity of gastric cancer cells through inhibition of proliferation and invasion via PI 3 K/AKT signaling pathway. Phytotherapy Research, 33(5), 1353-1362.
- Martin, A. C. B. M., Tomasin, R., Luna-Dulcey, L., Graminha, A. E., Naves, M. A., Teles,R. H. G., & Cominetti, M. R. (2020). [10]-Gingerol improves doxorubicin anticancer activity and decreases its side effects in triple negative breast cancer models. Cellular Oncology, 43(5), 915-929.
- Huang, Y., Xiao, D., Burton-Freeman, B. M., & Edirisinghe, I. (2016). Chemical changes of bioactive phytochemicals during thermal processing. Reference Module in Food Science, Elsevier, 77(9),1-6. DOI:10.1016/B978-0-08-100596-5.03055-9
- Choudhari, A. S., Mandave, P. C., Deshpande, M., Ranjekar, P., & Prakash, O. (2020). Phytochemicals in cancer treatment: From preclinical studies to clinical practice. Frontiers in Pharmacology, 10(1614), 1-17. DOI:https://doi.org/10.3389/fphar.2019.01614
- Quail, D. F., & Joyce, J. A. (2013). Microenvironmental regulation of tumor progression and metastasis. Nature Medicine, 19(11), 1423-1437.
- Arends, M. J. (2013). Pathways of colorectal carcinogenesis. Applied Immunohistochemistry & Molecular Morphology, 21(2), 97-102.
- Afrin, S., Giampieri, F., Gasparrini, M., Forbes-Hernández, T. Y., Cianciosi, D., Reboredo-Rodriguez, P., & Battino, M. (2020). Dietary phytochemicals in colorectal cancer prevention and treatment: a focus on the molecular mechanisms involved. Biotechnology Advances, 38(2020),1-38. DOI: 10.1016/j.biotechadv.2018.11.011.
- Lee, Y. C., Lin, H. H., Hsu, C. H., Wang, C. J., Chiang, T. A., & Chen, J. H. (2010). Inhibitory effects of andrographolide on migration and invasion in human non-small cell lung cancer A549 cells via down-regulation of PI3K/Akt signalling pathway. European Journal of Pharmacology, 632(1-3), 23-32.
- Rajagopal, S., Kumar, R. A., Deevi, D. S., Satyanarayana, C., & Rajagopalan, R. (2003). Andrographolide, a potential cancer therapeutic agent isolated from Andrographis paniculata. Journal of Experimental Therapeutics and Oncology, 3(3), 147-158.
- Imenshahidi, M., & Hosseinzadeh, H. (2019). Berberine and barberry (Berberis vulgaris): a clinical review. Phytotherapy Research, 33(3), 504-523.
- Wang, Y., Liu, Y., Du, X., Ma, H., & Yao, J. (2020). The anti-cancer mechanisms of berberine: a review. Cancer Management and Research, 12(1) 695-702. DOI: https://dx.doi.org/10.2147%2FCMAR.S242329
- G Gutheil, W., Reed, G., Ray, A., Anant, S., & Dhar, A. (2012). Crocetin: an agent derived from saffron for prevention and therapy for cancer. Current Pharmaceutical Biotechnology, 13(1), 173-179.
- Wang, C. J., Shiah, H. S., & Lin, J. K. (1991). Modulatory effect of crocetin on aflatoxin B1 cytotoxicity and DNA adduct formation in C3H10T12 fibroblast cell. Cancer Letters, 56(1), 1-10.
- Chryssanthi, D. G., Lamari, F. N., Iatrou, G., Pylara, A., Karamanos, N. K., & Cordopatis, P. (2007). Inhibition of breast cancer cell proliferation by style constituents of different Crocus species. Anticancer Research, 27(1A), 357-362.
- Giordano, A., & Tommonaro, G. (2019). Curcumin and cancer. Nutrients, 11(10), 2376.
- Wang, M., Jiang, S., Zhou, L., Yu, F., Ding, H., Li, P., & Wang, K. (2019). Potential mechanisms of action of curcumin for cancer prevention: focus on cellular signaling pathways and miRNAs. International Journal of Biological Sciences, 15(6), 1200.
- Sanders, B., Ray, A. M., Goldberg, S., Clark, T., McDaniel, H. R., Atlas, S. E., & Rasul, A. (2018). Anti-cancer effects of aloe-emodin: a systematic review. Journal of Clinical and Translational Research, 3(3), 283.
- Lissoni, P., Rovelli, F., Brivio, F., Zago, R., Colciago, M., Messina, G., & Porro, G. (2009). A randomized study of chemotherapy versus biochemotherapy with chemotherapy plus Aloe arborescens in patients with metastatic cancer. In Vivo, 23(1), 171-175.
- Ahmad, A., Padhye, S., & Sarkar, F. H. (2012). Role of novel nutraceuticals garcinol, plumbagin and mangiferin in the prevention and therapy of human malignancies: mechanisms of anticancer activity. In Nutraceuticals and Cancer (pp. 179-199). Springer, Dordrecht.
- Imran, M., Arshad, M. S., Butt, M. S., Kwon, J. H., Arshad, M. U., & Sultan, M. T. (2017). Mangiferin: a natural miracle bioactive compound against lifestyle related disorders. Lipids in Health and Disease, 16(1), 1-17.
- Cao, Y. Y., Yu, J., Liu, T. T., Yang, K. X., Yang, L. Y., Chen, Q., & Zhang, Y. (2018). Plumbagin inhibits the proliferation and survival of esophageal cancer cells by blocking STAT3-PLK1-AKT signaling. Cell Death & Disease, 9(2), 1-13.
- Zhang, X. Q., Yang, C. Y., Rao, X. F., & Xiong, J. P. (2016). Plumbagin shows anticancer activity in human breast cancer cells by the upregulation of p53 and p21 and suppression of G1 cell cycle regulators. European Journal, 37(1), 30-35. DOI: 10.12892/ejgo2765.2016
- Rafiq, R. A., Ganai, B. A., & Tasduq, S. A. (2015). Piperine promotes ultraviolet (UV)-B-induced cell death in B16F10 mouse melanoma cells through modulation of major regulators of cell survival. RSC Advances, 5(16), 11884-11894.
- Doucette, C. D., Hilchie, A. L., Liwski, R., & Hoskin, D. W. (2013). Piperine, a dietary phytochemical, inhibits angiogenesis. The Journal of Nutritional Biochemistry, 24(1),231-239.
- Kwak, T. W., Park, S. B., Kim, H. J., Jeong, Y. I., & Kang, D. H. (2017). Anticancer activities of epigallocatechin-3-gallate against cholangiocarcinoma cells. OncoTargets and Therapy, 10(1), 137-144.DOI: https://dx.doi.org/10.2147%2FOTT.S112364
- Min, K. J., & Kwon, T. K. (2014). Anticancer effects and molecular mechanisms of epigallocatechin-3-gallate. Integrative Medicine Research, 3(1), 16-24.
- Udenigwe, C. C., Ramprasath, V. R., Aluko, R. E., & Jones, P. J. (2008). Potential of resveratrol in anticancer and anti-inflammatory therapy. Nutrition Reviews, 66(8), 445-454.
- Varoni, E. M., Lo Faro, A. F., Sharifi-Rad, J., & Iriti, M. (2016). Anticancer molecularmechanisms of resveratrol. Frontiers in Nutrition, 3(8),1-15. DOI: https://dx.doi.org/10.3389%2Ffnut.2016.00008
- Preet, R., Chakraborty, B., Siddharth, S., Mohapatra, P., Das, D., Satapathy, S. R., & Chowdhury, C. (2014). Synthesis and biological evaluation of andrographolide analogues as anti-cancer agents. European Journal of Medicinal Chemistry, 85(2014), 95-106.
- Lim, J. C. W., Chan, T. K., Ng, D. S., Sagineedu, S. R., Stanslas, J., & Wong, W. F. (2012). Andrographolide and its analogues: versatile bioactive molecules for combating inflammation and cancer. Clinical and Experimental Pharmacology and Physiology, 39(3), 300-310.
- Tillhon, M., Ortiz, L. M. G., Lombardi, P., & Scovassi, A. I. (2012). Berberine: new perspectives for old remedies. Biochemical Pharmacology, 84(10), 1260-1267.
- Zhang, X., Gu, L., Li, J., Shah, N., He, J., Yang, L., & Zhou, M. (2010). Degradation of MDM2 by the interaction between berberine and DAXX leads to potent apoptosis in MDM2-overexpressing cancer cells. Cancer Research, 70(23), 9895-9904.
- Li, J., Gu, L., Zhang, H., Liu, T., Tian, D., Zhou, M., & Zhou, S. (2013). Berberine represses DAXX gene transcription and induces cancer cell apoptosis. Laboratory Investigation, 93(3), 354-364.
- Bathaie, S. Z., Hoshyar, R., Miri, H., & Sadeghizadeh, M. (2013). Anticancer effects of crocetin in both human adenocarcinoma gastric cancer cells and rat model of gastric cancer. Biochemistry and Cell Biology, 91(6), 397-403.
- Li, C. Y., Huang, W. F., Wang, Q. L., Wang, F., Cai, E., Hu, B., & Li, H. H. (2012). Crocetin induces cytotoxicity in colon cancer cells via p53-independent mechanisms. Asian Pacific Journal of Cancer Prevention, 13(8), 3757-3761.
- Mohan, C. D., Kim, C., Siveen, K. S., Manu, K. A., Rangappa, S., Chinnathambi, A., & Ahn, K. S. (2021). Crocetin imparts antiproliferative activity via inhibiting STAT3 signaling in hepatocellular carcinoma. IUBMB Life, 73(11), 1348-1362.
- Singh, S., & Khar, A. (2006). Biological effects of curcumin and its role in cancer chemoprevention and therapy. Anti-Cancer Agents in Medicinal Chemistry (Formerly Current Medicinal Chemistry-Anti-Cancer Agents), 6(3), 259-270.
- Wang, M., Jiang, S., Zhou, L., Yu, F., Ding, H., Li, P., & Wang, K. (2019). Potential mechanisms of action of curcumin for cancer prevention: focus on cellular signaling pathways and miRNAs. International Journal of Biological Sciences, 15(6), 1200.
- Bhatia, M., Bhalerao, M., Cruz‐Martins, N., & Kumar, D. (2021). Curcumin and cancer biology: Focusing regulatory effects in different signalling pathways. Phytotherapy Research, 35(9), 4913-4929. DOI:10.1002/ptr.7121.
- Thimmegowda, N. R., Park, C., Shwetha, B., Sakchaisri, K., Liu, K., Hwang, J., & Kim, B. Y. (2015). Synthesis and antitumor activity of natural compound aloe emodin derivatives. Chemical Biology & Drug Design, 85(5), 638-644.
- Guo, J., Xiao, B., Zhang, S., Liu, D., Liao, Y., & Sun, Q. (2007). Growth inhibitory effects of gastric cancer cells with an increase in S phase and alkaline phosphatase activity repression by aloe-emodin. Cancer Biology & Therapy, 6(1), 85-88.
- Dilshara, M. G., Kang, C. H., Choi, Y. H., & Kim, G. Y. (2015). Mangiferin inhibits tumor necrosis factor-α-induced matrix metalloproteinase-9 expression and cellular invasion by suppressing nuclear factor-κB activity. BMB Reports, 48(10), 559.
- Yin, Z., Zhang, J., Chen, L., Guo, Q., Yang, B., Zhang, W., & Kang, W. (2020). Anticancer effects and mechanisms of action of plumbagin: Review of research advances. BioMed Research International, 2020, Article ID 6940953, 1-10. DOI:https://doi.org/10.1155/2020/6940953
- Padhye, S., Dandawate, P., Yusufi, M., Ahmad, A., & Sarkar, F. H. (2012). Perspectives on medicinal properties of plumbagin and its analogs. Medicinal Research Reviews, 32(6), 1131-1158.
- Jamal, M. S., Parveen, S., Beg, M. A., Suhail, M., Chaudhary, A. G., Damanhouri, G. A., & Rehan, M. (2014). Anticancer compound plumbagin and its molecular targets: a structural insight into the inhibitory mechanisms using computational approaches. PLoS One, 9(2), e87309.
- Rather, R. A., & Bhagat, M. (2018). Cancer chemoprevention and piperine: molecular mechanisms and therapeutic opportunities. Frontiers in Cell and Developmental Biology, 6(10), 1-12. DOI:10.3389/fcell.2018.00010.
- de Almeida, G. C., Oliveira, L. F., Predes, D., Fokoue, H. H., Kuster, R. M., Oliveira, F. L., & Abreu, J. G. (2020). Piperine suppresses the Wnt/β-catenin pathway and has anticancer effects on colorectal cancer cells. Scientific Reports, 10(1), 1-12.
- Narayanan, S., Mony, U., Vijaykumar, D. K., Koyakutty, M., Paul-Prasanth, B., & Menon, D. (2015). Sequential release of epigallocatechin gallate and paclitaxel from PLGA-casein core/shell nanoparticles sensitizes drug-resistant breast cancer cells. Nanomedicine: Nanotechnology, Biology and Medicine, 11(6), 1399-1406.
- He, L., Zhang, E., Shi, J., Li, X., Zhou, K., Zhang, Q., & Tang, X. (2013). (−)-Epigallocatechin-3-gallate inhibits human papillomavirus (HPV)-16 oncoproteininduced angiogenesis in non-small cell lung cancer cells by targeting HIF-1α. Cancer Chemotherapy and Pharmacology, 71(3), 713-725.
- Tsai, H. Y., Ho, C. T., & Chen, Y. K. (2017). Biological actions and molecular effects of resveratrol, pterostilbene, and 3′-hydroxypterostilbene. Journal of Food and Drug Analysis, 25(1), 134-147.
- Xiao, Q., Zhu, W., Feng, W., Lee, S. S., Leung, A. W., Shen, J., & Xu, C. (2019). A review of resveratrol as a potent chemo protective and synergistic agent in cancer chemotherapy. Frontiers in pharmacology, 9(1534), 1-10 DOI:10.3389/fphar.2018.01534